In space exploration, the spectacle often outshines the scientific endeavor. Yet, regarding SpaceX's Starship and its unique landing maneuver, the "belly flop," the spectacle, and the science are inextricably linked. This audacious maneuver, where the mammoth rocket descends from the sky horizontally before executing a last-minute flip to land vertically, has been the subject of intense scrutiny and debate. Is it an engineering marvel, pushing the boundaries of what we thought possible? Or is it a safety concern, an unnecessary risk in an already high-stakes game?
Starship and its Belly Flop Maneuver
Imagine a skyscraper tumbling from the sky. That's the spectacle of SpaceX's Starship, a colossal rocket standing 50 meters tall and 9 meters wide, as it performs its signature belly flop maneuver. This daring descent sees the rocket plummeting through Earth's atmosphere, belly first before it spectacularly flips upright to stick a vertical landing. It's a thrilling sight, but it's also a source of heated debate among experts and enthusiasts alike.
The belly flop and flip maneuvers pose two essential questions: are they safe, and are they effective? Starship's ambitious design aims to revolutionize space travel, but these maneuvers pose serious challenges. The flip maneuver performed so close to the ground, leaves little room for error. The safety of human occupants is paramount, and the risk factor associated with this audacious maneuver cannot be ignored.
Aspects Affecting Starship's Landing Maneuver
The landing maneuver of the Starship is a complex ballet, influenced by various factors. Among these are the Engine Combinations used during landing. Starship employs a specific combination of its Raptor engines to control its descent, a critical aspect of the maneuver.
Then there's Terminal Velocity, the maximum speed the rocket can achieve while falling. It's a delicate balance: too fast, and the rocket risks a hard landing, too slow, and it might not have enough momentum to complete the flip.
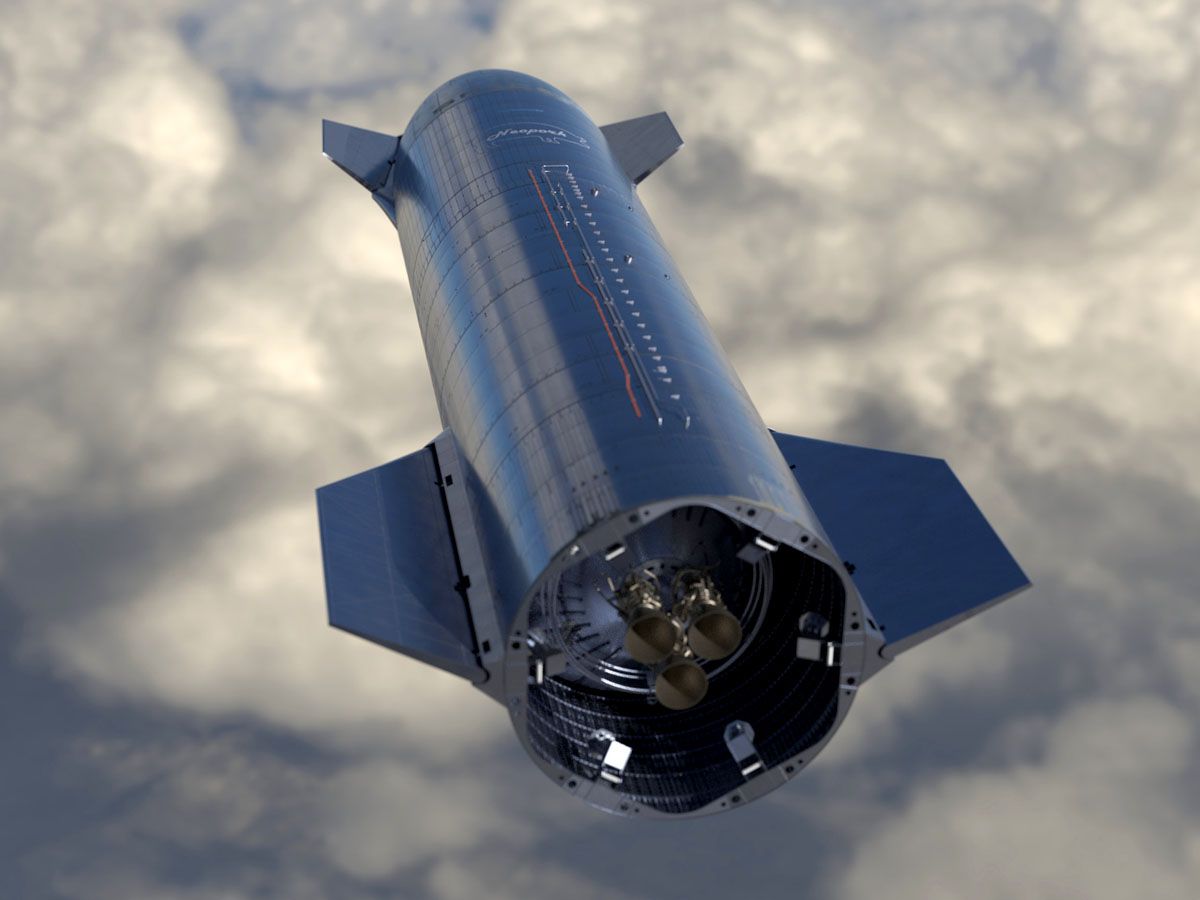
Gravity Drag, the resistance created by Earth's gravitational pull, and the thrust-to-weight ratio, a measure of how the rocket's thrust compares to its weight, also play crucial roles. These factors determine how effectively the Starship can counteract Earth's gravity to maintain control during its descent.
Lastly, Engine Throttling, the process of controlling the rocket's thrust, is a critical part of the landing equation. Too much thrust and Starship could overshoot its landing, too little, and it might not make it to the landing pad at all.
The Transition from Belly Flop to Tail Down
The transition from the belly flop to the tail-down position is a feat of engineering that seems to defy the laws of physics. When Starship reaches about 500 meters in altitude, it initiates this transition. Two Raptor engines roar to life, folding the rear flaps and swinging the rocket into a vertical position. Simultaneously, the rear fins retract, causing a rotation of the tail section under the nose.
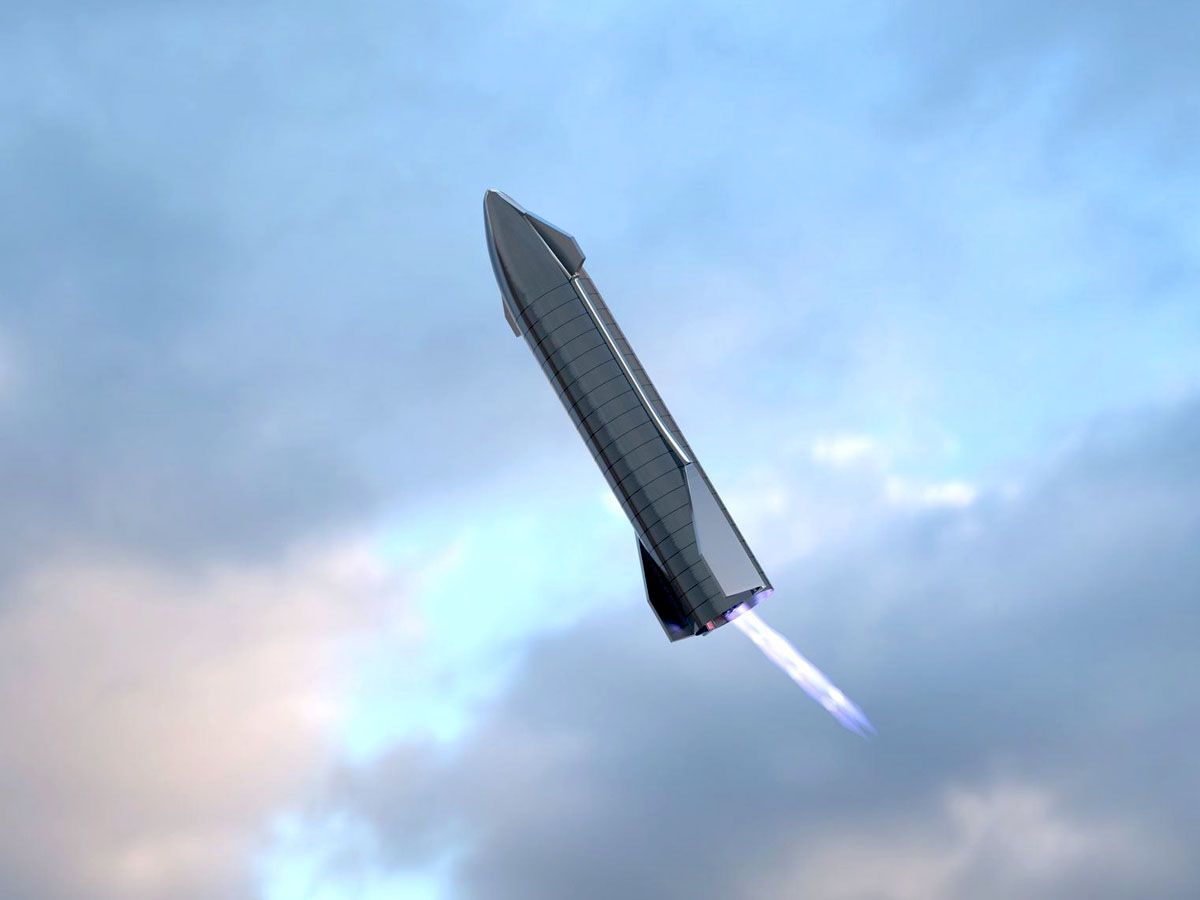
SpaceX plans to add hot gas thrusters in the future to improve the maneuver's efficiency and safety. These thrusters, powered by rocket propellant, will provide additional control during the flip and landing phases.
Propellant Settling on Starship's Belly
Another challenge that Starship faces during the belly flop maneuver is the settling of propellants on the belly side of the rocket due to atmospheric deceleration. This phenomenon can starve the engines of fuel, potentially leading to engine failure. To avoid this, Starship's engines draw propellant from header tanks, smaller tanks separate from the main propellant tanks.
These header tanks are strategically positioned in the nose portion and between the main tanks, ensuring that the engines have a steady supply of propellant, even while the rocket is falling belly-first through the sky. This ingenious solution showcases SpaceX's commitment to overcoming the unique challenges posed by the belly flop maneuver.
Terminal Velocity during a Rocket Landing
In the realm of rocket landings, managing speed is akin to threading a needle in gale-force winds. The terminal velocity defined as the constant maximum velocity that a falling object attains under the influence of gravity becomes a critical factor. It is within this complex dance of physics that the Starship's belly flop maneuver comes into play, using the spacecraft's substantial surface area as an atmospheric brake to decelerate its descent.
Starship capitalizes on its unique design to execute the belly flop maneuver. With a sprawling surface area of 545 square meters on its windward side, the side facing the direction of travel. Starship presents a substantial barrier to the rushing air during descent. Compare that to its base area, which is a mere 70 square meters, and the brilliance of the belly flop maneuver becomes apparent.
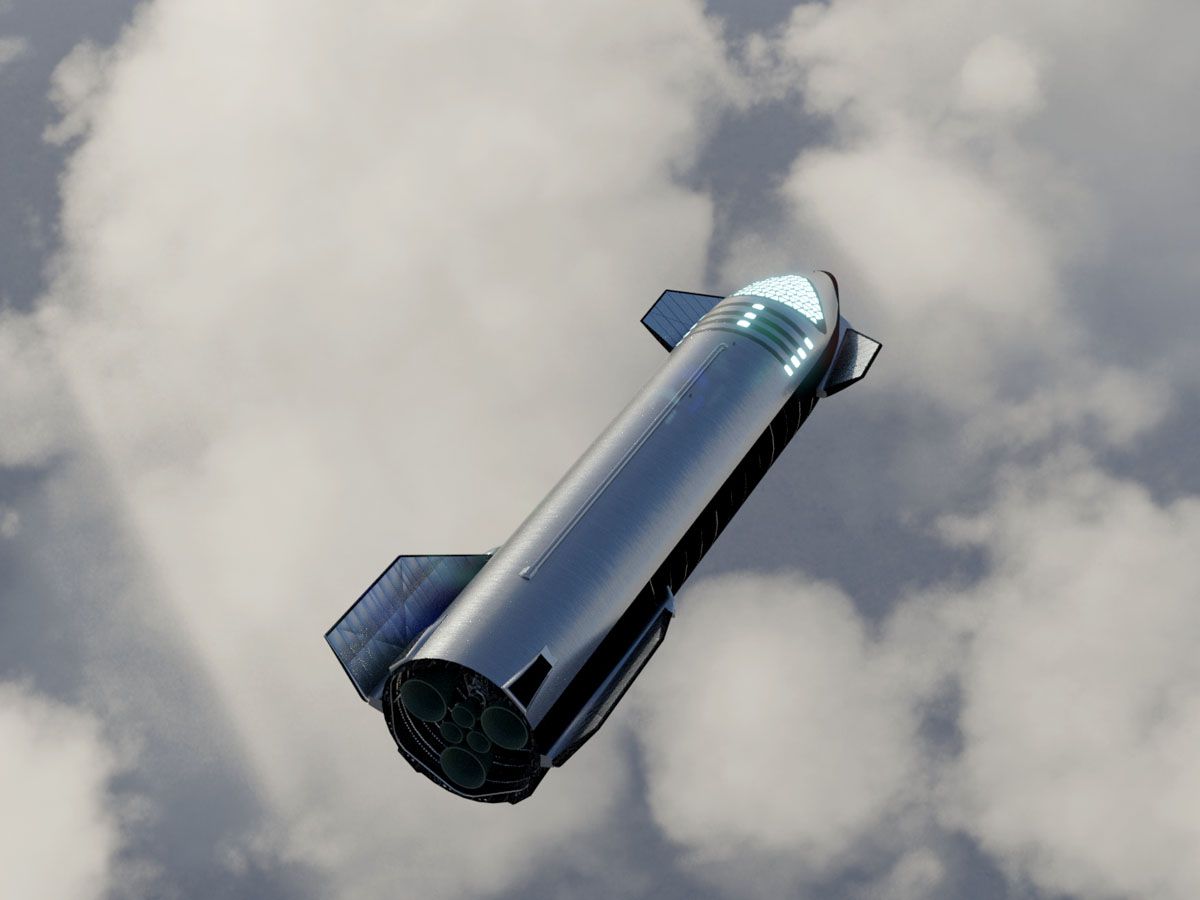
Starship significantly reduces its terminal velocity by effectively increasing its drag in this position. This deceleration is akin to a driver downshifting instead of using the brakes allowing the rocket to conserve fuel and initiate its landing burn later. The result is a greater margin of error for trajectory corrections and additional time to prepare for the all-important landing flip a testament to the genius of SpaceX's unconventional approach to spacecraft design.
How does this compare to SpaceX's other workhorse, the Falcon 9? Falcon 9 descends at a faster rate due to its sleeker, more streamlined design. It has less surface area to generate drag, resulting in a higher terminal velocity. On the other hand, Starship's flaps play a crucial role in controlling its terminal velocity, thanks to its larger surface area exposed during the belly flop.
Thrust to Weight Ratios
In rocket science, maintaining a 1:1 thrust-to-weight ratio is akin to finding the perfect balance. The rocket needs this balance to hover. Any less and it falls, any more and it ascends. Even minor fluctuations in this ratio can significantly affect the rocket's velocity during descent and landing.
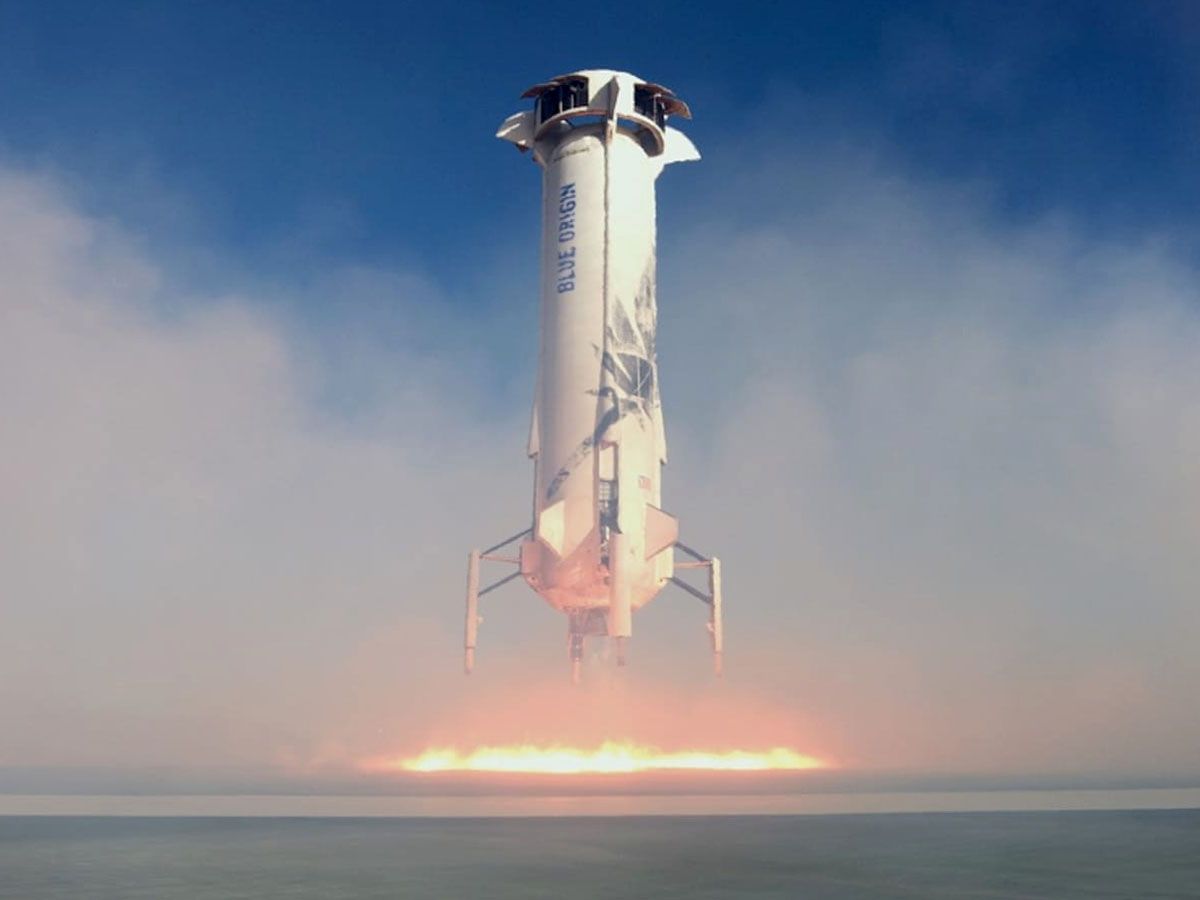
For instance, an increase in the thrust-to-weight ratio can cancel out the rocket's downward velocity. This change allows the rocket to slow down and eventually hover, facilitating a controlled landing. However, managing this ratio is a tricky endeavor and requires precision engineering and real-time adjustments.
Throttling on Landing
To manage the delicate thrust-to-weight ratios, rockets employ a technique called throttling. Throttling is the process of adjusting the rocket's thrust in real-time, a critical factor during the landing phase.
How does throttling relate to the thrust-to-weight ratios? Simply put, by adjusting the throttle, rockets can control their thrust and, therefore, their thrust-to-weight ratios. This control allows them to manage their descent speed and successfully land.
For comparison, consider the Falcon 9, which lands at a throttling level of around 70 percent. This throttle setting gives the rocket enough thrust to counteract gravity and achieve a soft landing. However, Starship's throttle settings might differ due to its unique design and belly-flop maneuver, highlighting the complexity and diversity of SpaceX's rocket engineering.
Gravity Loss during Rocket Landing
Gravity loss is a key consideration during rocket landings. This phenomenon occurs when a rocket fights against gravity to maintain its position or alter its trajectory, resulting in a loss of potential forward motion, or 'delta-v'. Essentially, the more a rocket fights gravity, the more fuel it consumes, leading to a higher gravity loss.
The thrust-to-weight ratio plays a significant role in determining the extent of gravity loss. A higher ratio means less propellant is wasted in fighting gravity, hence reducing gravity loss. The balance between maximizing efficiency and minimizing fuel consumption requires a meticulous calibration of this ratio.
Starship Flipping at Different Altitudes
SpaceX's Starship introduces a unique aspect to rocket landing: flipping at different altitudes. The decision to flip close to the ground has its merits. The primary benefit is that it reduces the terminal velocity, allowing the Starship more control over its descent and landing.
The flip maneuver's execution requires an intricate dance of engines. Starship's Raptor engines have a broad throttling range, between 40% and 100%, providing the flexibility required for this maneuver. Depending on the altitude and velocity, different engine combinations are utilized to execute the flip.
However, the altitude at which the flip starts significantly affects the delta-v requirements. A higher flip altitude may provide more room for error correction, but it increases the delta-v requirements, impacting fuel consumption and efficiency.
Crew during Flip Maneuver
As SpaceX sets its sights on human missions to Mars, the crew's experience during the flip maneuver is of paramount importance. The Raptor engine, still in development, needs to demonstrate high reliability to ensure the crew's safety during this critical phase of the mission.
One of the main concerns is the G forces experienced during the flip maneuver. The peak G force during this maneuver is estimated to be around 2.5 G's, a force comparable to some of the most intense roller coasters. This stress could be physically demanding and potentially disorientating for the crew.
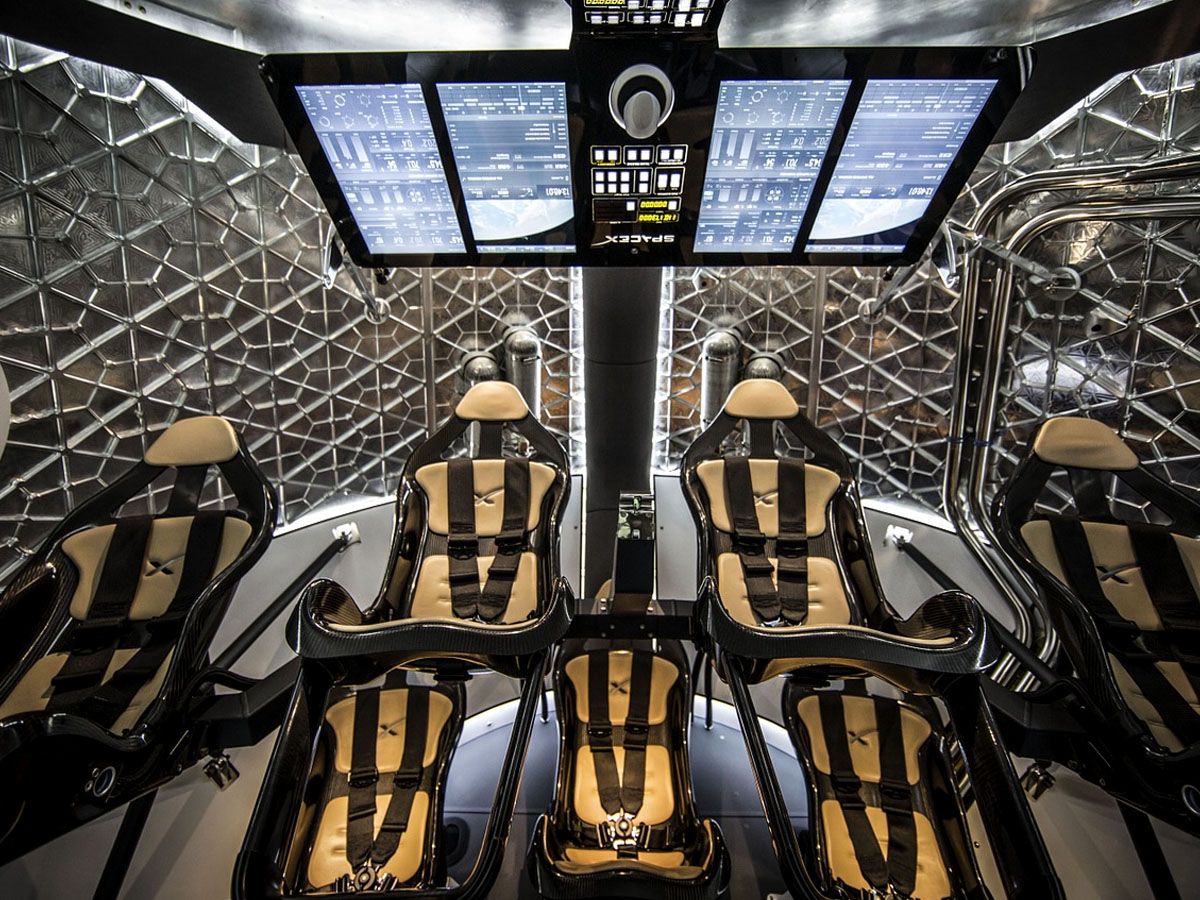
SpaceX is exploring potential solutions to mitigate these forces. One option could be rotating seats that move to align with the force direction, reducing the perceived impact on the crew. While the technological challenges are formidable, the potential payoff, a new era of interplanetary human exploration is well worth the effort.
Sources: everydayastronaut.com / futurism.com / oxbridgelaunchpad.com